Highlight
2018.08.22 “Hole in One” of Nanoparticles into Gold Nano-Gaps
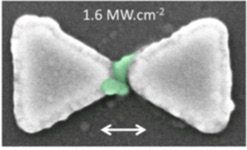
Fig. 1 SEM micrographs of fluorescent nanoparticles optically trapped and deposited in a nano-gap of gold antenna (the side length of the gold triangles is 250 nm).
Keiji Sasaki
(Planned Research A03: Hokkaido University)
In our collaboration research with Prof. Fukaminato’s group, we developed a novel technique for trapping and deposition of colloidal nanoparticles in nanometer-sized gaps of gold structures. In this technique, the gold nano-gap structure is prepared by cutting edge nano-fabrication technology, and a laser light is confined to form extremely intense nano-spot in the gap (the field enhancement reaches to 10,000), that is the super-focusing lens effect of the metal nano-gap. The strongly localized field induces so strong optical force that it can attract nanoparticles dispersed in liquid and can trap them in the gap without mechanical contacts. In addition, temperature elevation of the gold nanostructure by laser irradiation makes it possible to fix and deposit the trapped nanoparticles in the gap. In the demonstration experiment, we succeeded in one-step optical process for trapping and deposition of brightly emitting organic molecule nanoparticles prepared by Prof. Fukaminato (Fig. 1). The scanning electron microscope images showed that the molecular particles with few tens of nanometer sizes were fixed in the gap of the gold bowtie antenna. This proposed technique is applicable to hybrid plasmonic components, molecular electronic devices, functional nanophotonic systems, and so on.
(1) C. Pin, T. Fukaminato, K. Sasaki, et al., ACS Omega, 2018, 3, 4878-4882.
2018.07.25 Molecular manipulation at the strong coupling states
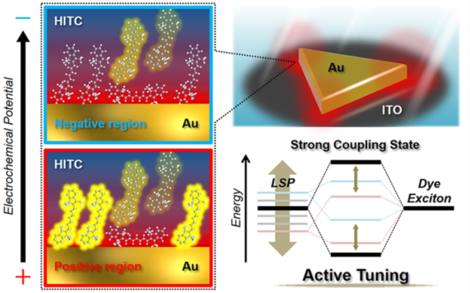
Fig. 1 Schematic illustration of the molecular manipulation at the strong coupling states between localized surface plasmons and dye excitons under electrochemical potential control.
Kei Murakoshi
(Planned Research A03: Hokkaido University)
The strong coupling state is the electronic interaction between the light energy and the excition of the dye molecule at the surface of the plasmon active structure. This electronic interaction leads to the formation of the new hybridized energy state which allows the system to expand the response wavelength region. Based on this effective light energy usage, it can be expected that the efficient optical molecular manipulation would be realized in the small cavity. In our recent study, we have successfully tuned the coupling strength of the strong coupling state by the electrochemically control of the plasmon resonance energy. In the potential region more negative than the reduction potential of the dye molecule, the coupling strength decreased due to the reduction reaction of the molecules. One of the interesting point for this study is that the coupling strength gradually increased by the electrochemical potential scan from positive potential region to the reduction potential. This could be originated from that the distance between the molecule and the surface of the metal became closer by the effect of the light energy. Our present achievements strongly imply the possibility for the realization of the optical manipulation in the strong coupling state where the light energy is efficiently transferred to the molecules.
(1)F. Kato, H. Minamimoto, and, K. Murakoshi et al., ACS Photon., 2018, in press. doi: 10.1021/acsphotonics.7b00841
(2) H. Minamimoto, F. Kato,and, K. Murakoshi et al., Faraday Discuss., 2017, 205, 261
2018.04.30 Coalescence of microdroplets by optical trapping
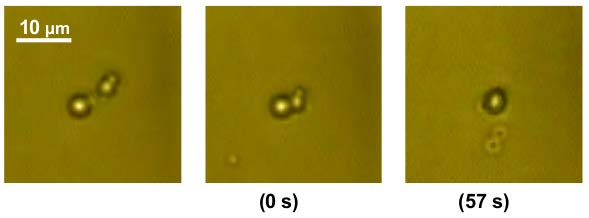
Fig. 1 Coalescence of oil droplets manipulated by optical trapping. The numbers in parentheses indicate the time after contacting two droplets.
Takashi Kaneta
(Publicly Offered Research: Okayama University)
Optical trapping using optical forces is known to be a manipulation technique of small objects. The optical trapping techniques allow the non-contact and selective manipulation of the small objects with less damage in contract to conventional manipulation techniques. Therefore, the techniques can be employed in the manipulation of biological cells and cell organelles that are easily destructed by an external stimulus. We developed a method to coalesce two micro-droplets by manipulating them independently using two laser beams toward chemical analyses of the components in a single cell and a single droplet (1).
In this study, we tried to merge two oil droplets dispersed in water using optical trapping. In general, oil droplets are stabilized by a surfactant in water in order to hydrophilize the surface of oil droplets so that the surfactant molecules adsorbed on the droplets prevents the coalescence of the oil droplets. When using cationic, anionic, and electrically-neutral surfactants, the hydrophilic nature of electrically-neutral surfactants made it a useful emulsifier for the coalescence of the oil droplets. Furthermore, it was found that the increase in temperature activated the molecular motion of the surfactant on the oil droplets, resulting in the coalescence of the oil droplets. The time required for the coalescence decreased with increasing temperature and the activation energy was estimated to be 4 kJ mol-1.
The present study demonstrated utility and applicability of optical pressure for biochemical analyses in a micro space, so the results indicated the social significance of the Research on Innovative Area, “Nano-Material Manipulation and Structural Order Control with Optical Forces”. Furthermore, the present study will be extended to the research on the collection of nano-vesicles. Our final goal is to apply the technique using optical forces to the collection and analyses of the exosomes that are released from cancerous cells for early diagnosis of cancers.
(1) M. Mitsunobu, S. Kobayashi, N. Takeyasu, T. Kaneta, Anal. Sci., 2017, 33, 709–713.
2018.03.20 Nano-optofluidics: Coupling Nanofluidics with Optical Forces
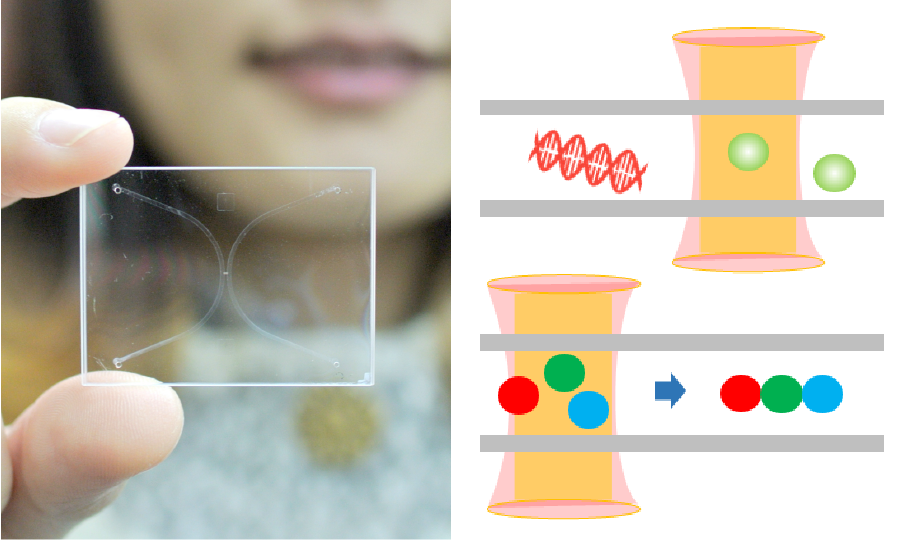
Fig. 1 Nanofluidic devices (left) coupling with optical manipulation allow the precise arrangement of single nanoparticles.
Yan Xu
(Publicly Offered Research: Osaka Prefecture University)
Current understanding of nanoscale transport and knowledge of physics, biology, and materials science allow us to envision new mechanisms and novel techniques to achieve fast, high throughput manipulation of individual nanoscale objects—whether biological or nonbiological entities—with superhigh spatial–temporal resolution, rather than masses of all at once. To reach this goal, the considerable development of technology is required. Nanofluidic technology (Fig. 1, left) is of course necessary. This should come as no surprise, considering that the dimensions of nanochannels approach the dimensions of many biological and nanoscale objects of interest in a wide range of disciplines (1).
By utilizing nano-in-nano integration technology (2), we coupled nanofluidics with optical manipulation for the first time to develop powerful tools enabling complex manipulation and precise arrangement of single nanoparticles in the liquid phase (Fig. 1, right), which is greatly challenging in the field of optical manipulation. Our recent progress in this evolved optofluidics (called “nano-optofluidics” or nano-in-nano optofluidics” by us) holds the potential to achieve high-throughput precise arrangement of single nanoparticles over a macroscopic region, which is also an undergoing study of our group.
(1) Y. Xu, Adv. Mater., 2018, 30, 1870019.
(2) Y. Xu et al., Lab Chip, 2015 , 15, 1989-1993.
2017.12.20 Elucidating the Mechanism of Particle Transportation due to Photoinduced Convective Flow Toward Efficient Assistance for Resonant Optical Manipulation
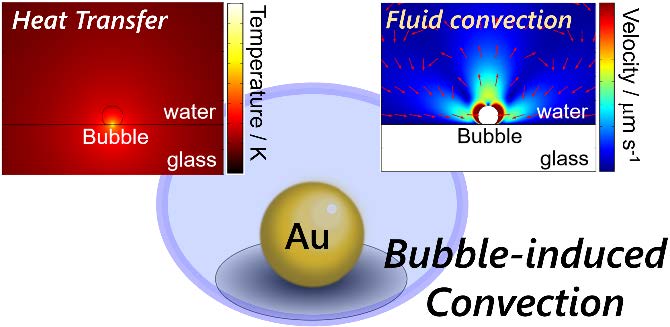
Fig. 1 Convective flow induced by photo-thermal conversion of single gold nanoparticle
Syoji Ito
(Planned Research A02: Osaka University)
We are developing methods to control radiation force acting on small materials on the basis of the change of their photo-response, such as absorption and scattering cross sections, by using photochemical reactions. As the momentum of photon can be resonantly transferred to material trough resonant photo-absorption, photoirradiation at a resonant wavelength leads to the generation of strong absorption force. In particular, metal nanoparticles show significant near-field antenna effect due to localized surface plasmon resonance (LSPR); as strong radiation force can be expected around metal nanoparticles showing LSPR, they are regarded as a key of nanoscale optical manipulation. However, the absorbed photon-energy is usually converted to heat, leading to mass-flow due to thermal convection and thermophoresis. As the photothermally induced mass transfer is expected to assist/prevent controlled manipulation, it is indispensable to quantitatively elucidate and control the photothermal effect for precise optical nano-manipulation.
For this purpose, we have experimentally and numerically investigated photothermal convection caused by gold nanoparticles under intense photoexcitation in water.(1) Photo-irradiation of a gold nanoparticle with 150-nm diameter at 532 nm led to the formation of a microbubble around the particle ranging sub to several micrometers depending on excitation intensity. Accompanying the bubble formation, detectable convective flow was observed. We conducted numerical simulations for heat and flow fields. Comparing the numerical and experimental results we concluded that the convective flow caused by photoexcitation of single gold nanoparticle is governed by Marangoni convection and natural convection is negligible. The information obtained through the study will contribute to an efficient cooperative optical nano-manipulation with photothermal convective flow.
(1) K. Setoura, S. Ito, H. Miyasaka, Nanoscale, 2017, 9, 719-730.
2017.11.30 Direct measurement of optical pressure by using carbon nanotube mechanical resonator
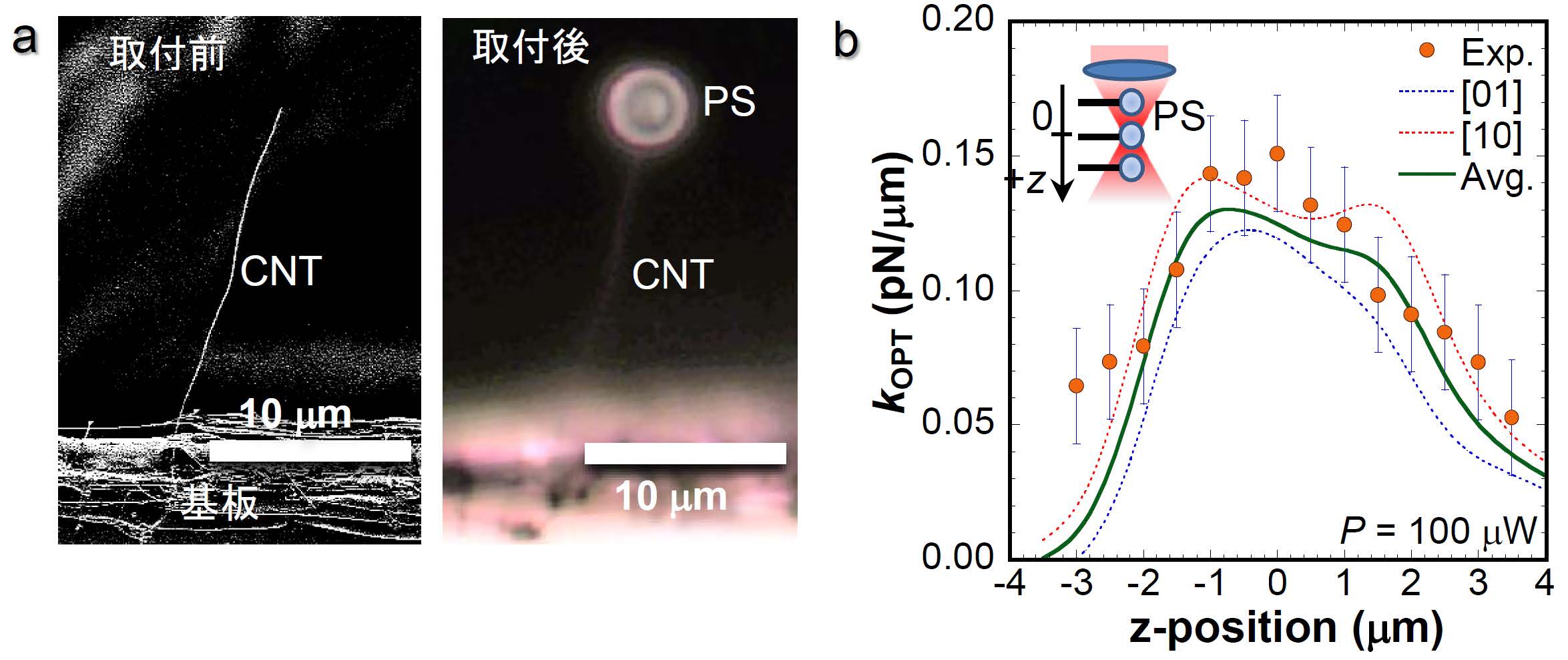
Fig. 1(a) CNT mechanical resonator with a PS beads and (b) Distribution of optical pressure gradient working on the PS beads.
Seiji Akita
(Planned Research A01: Osaka Prefecture University)
Carbon nanotubes (CNTs) are applicable to ultra-sensitive force sensors capable of measuring masses on the order of 10^-21 g, because of their excellent mechanical resonance characteristics and extremely light weight less than 10^-15 g. Using this highly sensitive force sensor, direct measurement of the distribution of light pressure acting on polystyrene (PS) beads, which is widely used for measurement of biological samples by optical tweezers, was investigated (1). While measurement of optical pressure acting on PS particles has been mainly analyzed in liquid, we measured the optical pressure in vacuum using CNT mechanical resonator.
In order to realize this, it is important to attach the PS beads to the tip of the CNT with good reproducibility. In this study, the CNT was heated with a laser under the optical microscope observation and PS beads were welded to the CNT tip in the air by micromanipulation (Fig. 1a). The diameter of the PS beads is about 3 μm. The mass of PS beads obtained from the change in the resonance frequency of the CNT mechanical resonator was 13 pg. This is approximately equal to the mass obtained from its diameter and density. Thus, the CNT cantilever acts as a mechanical resonator even when the PS particle are attached to the CNT tip.
The spatial distribution of the force gradient of optical pressure working on the PS beads was measured by changing the focal position of the light. The detection limit of the optical force gradient was determined to be 0.02 pN/μm, which is close to the thermal noise limit. In order to suppress photoinduced thermal effects, the light intensity was set to 100 μW, which is lower than the intensity used in liquid. Figure 1b shows the force gradient distribution in the focal depth direction. The measured results are in good agreement with the prediction by the theoretical calculation. Our proposed method can be easily transferred in an ideal environment with extremely low disturbance of heat and viscosity such as cryogenic temperature and ultrahigh vacuum, so that this direct measurement can contribute to further elucidation of the optical pressure acting on particles of nanometer scale.
(1) M. Yasuda et al., Scientific Reports, 2017, 7, 2825.
2017.11.16 Optical fabrication of ZnO microspheres in superfluid helium and their inner structures
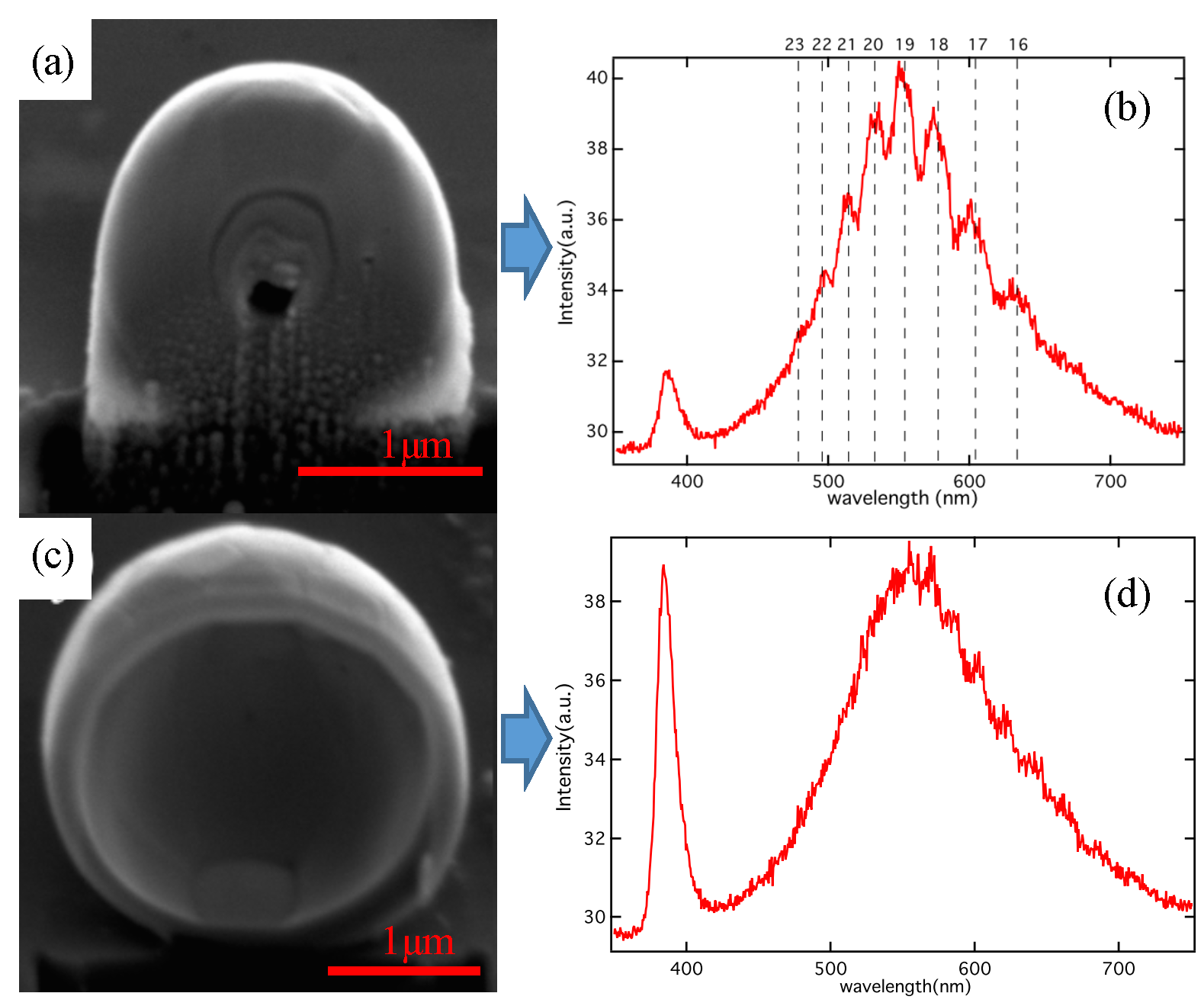
Fig. 1 SEM images of ZnO microspheres cutted by FIB (a) and (c) and their luminescence spectra (b) and (d), respectively. The lengths of the bars correspond to 1 µm. The WGMs were observed in (b), where the mode numbers are also shown.
Masaaki Ashid
(Planned Research A02: Osaka University)
Microspheres with diameter larger than light wavelength work as optical resonators, which are called whispering gallery modes (WGMs) resonators. In particular, semiconductor microspheres, which work as light emitters along with the resonators, have a high potential in the application of micro-lasers. However, high sphericity and crystallinity are difficult to be satisfied simultaneously, because crystals have facet planes. The fabrication of anisotropic semiconductors, such as ZnO, has been thought to be almost impossible. On the other hand, surprisingly, we succeeded in the fabrication of highly-spherical and single-crystalline ZnO microspheres by laser ablation in superfluid helium (1). Moreover, we found laser oscillation of WGMs in the fabricated ZnO microspheres with very low excitation threshold (2). Using this novel method, we successfully fabricated many nano- and micro spheres of different materials, such as CdSe, ZnSe, etc. (3).
In the project, “nano-material manipulation and structural order control with optical forces,” we are performing the experiments of the optical manipulation of such nano- and micro particles in superfluid helium having extremely low temperature and viscosity. Here the inner structures of the fabricated spheres are crucial to analyze the motion under optical forces. Thus we are now conducting the observation inside the microspheres, where transmission electron microscopy is not available due to the large thickness of the spheres, using a focused ion beam (FIB) system which enables us to cut the spheres. Figures 1 (a) and (c) show typical examples of the inner structures of the microspheres (4). In both cases voids were found, but the luminescence spectra showed different features as follows: In Fig. 1 (b), the spectrum of the sphere in Fig. (a), WGMs were clearly observed so that the optical property is not significantly affected by the void. On the other hand, in Fig. 1 (d) corresponding to the sphere whose inner structure resembles a spherical shell as seen in Fig. 1 (c), WGMs completely disappeared.
(1) S. Okamoto, M. A. et al., Sci. Rep., 2014, 4, 5186.
(2) S. Okamoto, Y. Minowa and M. A.,Proc. of SPIE, 2012, 8263, 82630K.
(3) S. Okamoto, S. Ichikawa, Y. Minowa and M. A., MRS Online Proceedings Library, 2015, 1736, mrsf14-1736-t08-02.
(4) Y. Minowa, Y. Oguni, M. A., Opt. Exp., 2017, 25, 10449.
2017.11.13 High-sensitive and high-resolution imaging of the photo-induced force without the photo-thermal effect
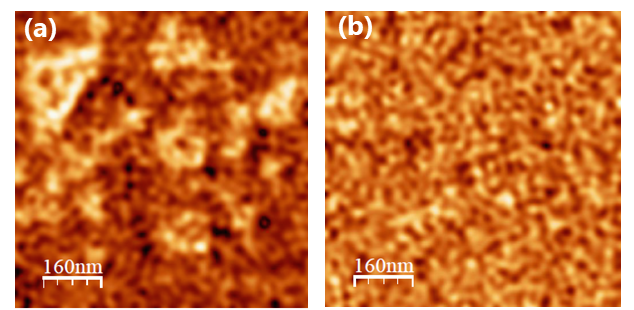
Fig.1 Photo-induced force images of the Au thin film surface using (a) heterodyne-FM and (b) heterodyne-AM techniques.
Yasuhiro Sugawara
(Planned research A01:Osaka University)
We make plans to measure the photo-induced force acting on nanomaterials with high sensivitity and high spatial resolution using atomic force microscopy (AFM), which has high force sensitivity and high spatial resolution. When the nanomaterial is illuminated by the amplitude-modulated laser light and the modulated conponent of the force acting the AFM tip is measured, not only the photo-induced force but also the photo-thermal force due to the photo-thermal effect for the AFM tip are simultaneously detected, because both of the nanomaterial and the AFM tip are illuminated by the laser light. The method for separating the photo-induced force from the photo-thermal force should be developed.
In this project, we have investigated a new method for separating the photo-induced force from the photo-thermal force. First, we have proposed the heterodyne-amplitude-modulation (heterodyne-AM) technique and demonstrated the inhibition of the influence of the photo-thermal force [1]. In this technique, the sensitivity of the photo-induced force is not suficient because the photo-induced force is measured using the oscillating amplitude of the second resonance frequency of the cantilever with the high spring constant. Then, we have proposed the heterodyne-frequency-modulation (heterodyne-FM) technique to enhance the sensivitity of the photo-induced force [2]. In this technique, the sensitivity of the photo-induced force is strongly improved, because the FM technique with high force sensitivity is used and the photo-induced force is measured using the frequency shift of the first resonance frequency of the cantilever with the low spring constant. Figures 1(a) and 1(b) represent the photo-induced force images of the Au thin film surface using heterodyne-FM and heterodyne-AM techniques, respectively. We suceeded in clearly observing the distribution of the photo-induced force on the surace with 10 nm spatial resolution. We will measure the wavelength dependence of the photo-induced force for quantum dots with the quntized levels in the future.
(1) J. Yamanishi, Y. Naitoh, Y. J. Li and Y. Sugawara, Appl. Phys. Lett., 2017, 110, 123102.
2017.11.12 Dispersion and isolation of semiconductor quantum dots in buffer gases
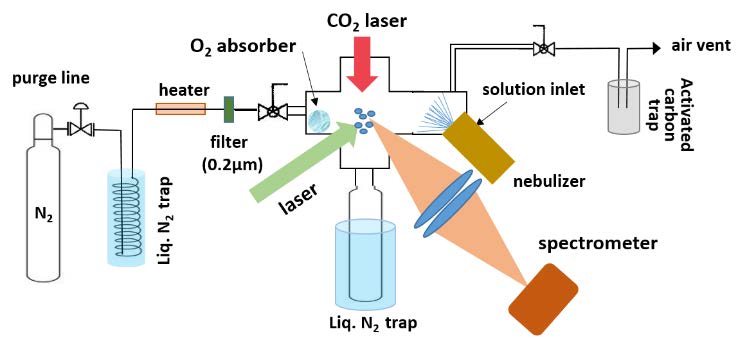
Fig.1 Experimental setup
Mitsutaka Kumakura
(Planned Research A02: University of Fukui)
Since optical properties of semiconductor quantum dots (QDs) with diameters of a few nanometers greatly depend on their particle sizes, size separation is expected as one of important processes for future applications of chemically synthesized QDs, which are generally composed of various particle sizes, for optical devices with high efficiency and/or coherence. The aim of this work is to realize the nondestructive dispersion and isolation of such nanoparticles in buffer gases and to experimentally explore the possibility of their size separation by optical manipulation.
Figure 1 shows our experimental setup developed for the nondestructive dispersion and isolation of CdSe/ZnS core-shell type QDs. The droplet of the toluene solution of the QDs are dispersed into a dry nitrogen gas with a nebulizer using a piezoelectric transducer. The size of the generated droplet is typically 5 µm approximately. The motion of the droplet is monitored by the imaging of the scattered laser light. For isolating the QD in the buffer gas, the organic solvent is removed from the droplet by CO2 laser heating. Moreover, to enhance the evaporation, the gas cell is heated to nearly 323 K and the solvent vapor is eliminated with a cold trap using liquid nitrogen. The evaporation of the droplet is monitored by observing the scattering intensity of the probe laser beam, and it was confirmed that almost all droplets evaporated in roughly 30 s. The change of the optical properties of the QD is also monitored through the isolation process by observing the fluorescence spectrum from the QDs; for example, the peak shift of the fluorescence spectrum due to the temperature rise during the CO2 laser heating was confirmed experimentally.
For increasing the QD density greatly, we are also working on the development of another experimental apparatus using much smaller clusters with sizes of several tens of nanometers. These unconventional experimental conditions for nanoparticles are expected to be valuable as a novel experimental environment for the optical manipulation experiment because of the wide controllability of the temperature, low viscosity, and so on. For example, since quantum nature generally plays a more significant role in the optical property of nanoparticles at a cryogenic temperature, the physical system of nanoparticles in a cold buffer gas should be useful for their optical manipulation using discrete quantum levels and their coherence.
2017.10.26 Microscopic Imaging with Circular Dichroism
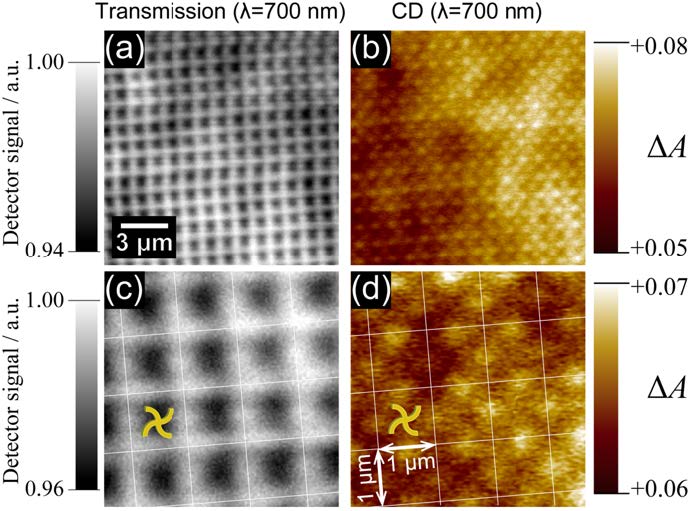
Figure 1. Optical transmission microscopic images (a,c) and circular dichroism images (b,d) of swirl-shaped gold nanostructure array. (1)
Hiromi Okamoto
(Planned Research A02, Institute for Molecular Science)
A material is chiral when the mirror-imaged structure of it is not superposable to its original one. Many molecules have chiral structures. Particularly, most of molecules that compose biological systems are chiral. Circular dichroism (CD) is differential optical absorption intensities of materials between left- and right-handed circularly polarized light. Chiral materials show CD, while achial (non-chiral) materials do not. Because of this feature, CD is widely utilized to judge whether the material is chiral, in particular for liquid and solution samples.
CD imaging of samples with microstructures has much potential in revealing chiral materials distribution, polymorphism of crystals, functions of materials, and so forth. The signal amplitude of CD is, however, usually very small, and the signal is sometimes strongly interfered by other polarization characteristics such as optical anisotropy of solid-state materials. Reports on CD microscopic imaging is very limited for this reason. In the present study, we proposed a novel method of CD measurement that is in principle not affected by those interference factors, and developed an apparatus that achieved high-precision CD microscopic imaging measurements.
With this apparatus we measured CD imaging of 2-dimensional gold nanostructure samples fabricated by the electron-beam lithography and lift-off technique, and found that CD images of sufficiently good quality were obtained. Interestingly, the spatial resolution of the observed CD images was apparently higher than that determined by the diffraction limit of light. This result is probably due to the bipolar character of CD signals (both positive and negative signals are possible), which is a different situation from ordinary intensity signal. The developed apparatus can be utilized for microscopic CD imaging of microstructured (down to sub-micrometer regime) samples. We expect that it may be useful for characterization of chiral crystals and nano- and micro-structured samples fabricated with optical forces.
(1) T. Narushima, H. Okamoto, Sci. Rep., 2016, 6, 35731.
(2) H. Okamoto, T. Narushima, Japanese PAT pending, 2017-120255.
2017.09.30 Designed synchronized-fluorescence of the emitter ensemble
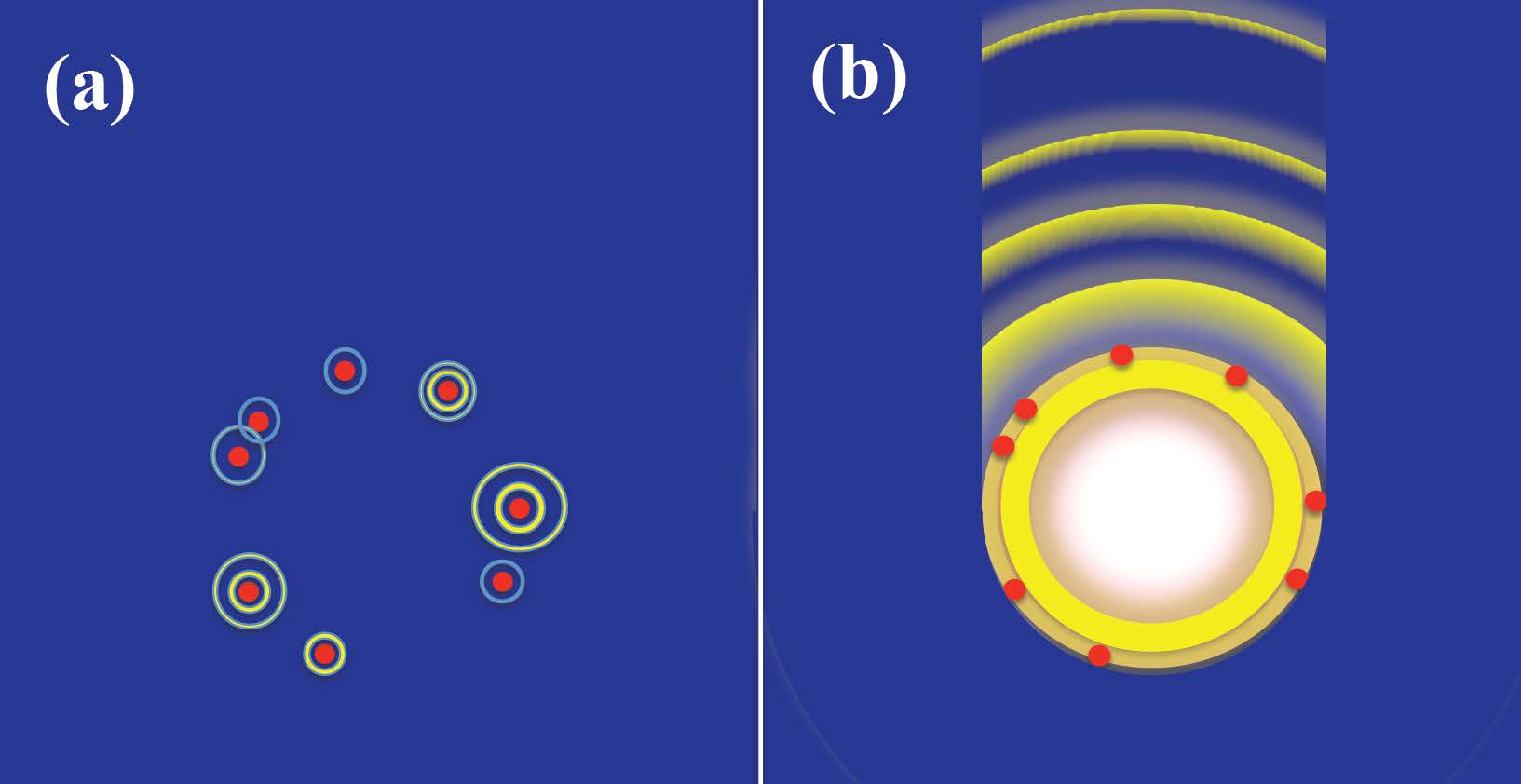
Fig. 1 Superfluorescence image of a dispersed molecular system. (a) The molecules are simply dispersed in a vacuum. When the molecular positions are sufficiently separated, each molecule emits a light independently. (b) The molecules are located on the dielectric sphere. The resonator mode in the sphere makes the well-separated molecules correlate with each other, and exhibit a directional superfluorescence.
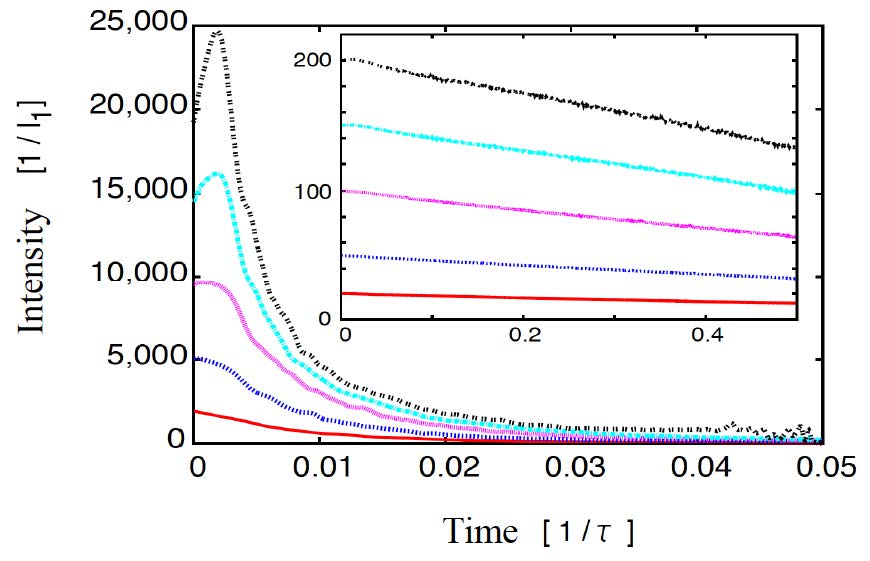
Fig. 2 Fluorescence intensity dynamics for a dispersed molecules. The number of molecules N is 20 to 200. I1 and τ are the intensity and radiation lifetime when N=1. The characteristic profile to cooperative superfluorescence clearly appears. The inset show the case where the molecules are set in a vacuum. The fluorescence exhibits a simple exponential decay and no cooperative phenomenon can be seen.
Hajime Ishihara
(Planned Research A01: Osaka Prefecture University)
In optical manipulation technique, linear optical responses of target materials usually determine the radiation pressure acting on them. On the other hand, in optical responses of materials, there are various scattering and absorption processes beyond linear response theory. The active usage of such processes has possibility to control the unconventional mechanical motion with higher degree of freedom. For example, a significant stimulated emission occurs when the population of the target material is inverted, i.e., the occupancy of the excited state exceeds that of the ground state. In this case, to preserve the total momentum including absorbed/emitted photons, a new dynamic action appears: the radiation pressure becomes attracted to the light source side (1). From this example, it is expected that we can design mechanical control of materials by diversifying light-matter coupling processes.
In this research project, we constructed a new theoretical model that describes a synchronized light emission of molecules in arbitrary geometric arrangement, and demonstrated superfluorescence of dispersed molecular system (2). It is known that superfluorescence, which is directional coherent light, is generated due to the inter-molecule correlations when the molecules exhibit population inversion. Using such a phenomenon for the control of the photoexcitation process, there is a possibility to realize unconventional optical manipulations. As an example, we investigated the synchronized photoemission of dye molecules that is sprayed on a dielectric microsphere. It was found that a significant superfluorescence phenomenon appears due to the intermolecular correlation even though the inter-molecule spacing exceeds the radiation wavelength (Fig. 1, 2).
The above result showed for the first time that the superfluorescence of molecules can be designed depending on their arrangement and their environment including the optical mode structure. In the future, by using such a mechanism, we will realize a novel mechanical action beyond simple momentum exchanges with incident light so that we increase the degree of freedom of optical manipulation. We will also clarify the relationship between radiation pressures and diversity of light-matter couplings.
(1) T. Kudo and H. Ishihara, Phys. Rev. Lett., 2012, 109, 087402
(2) N. Yokoshi, K. Odagiri, A. Ishikawa, H. Ishihara, Phys. Rev. Lett., 2017, 118, 203601
2017.09.29 Development of Non-Plasmonic NASSCA Optical Tweezer
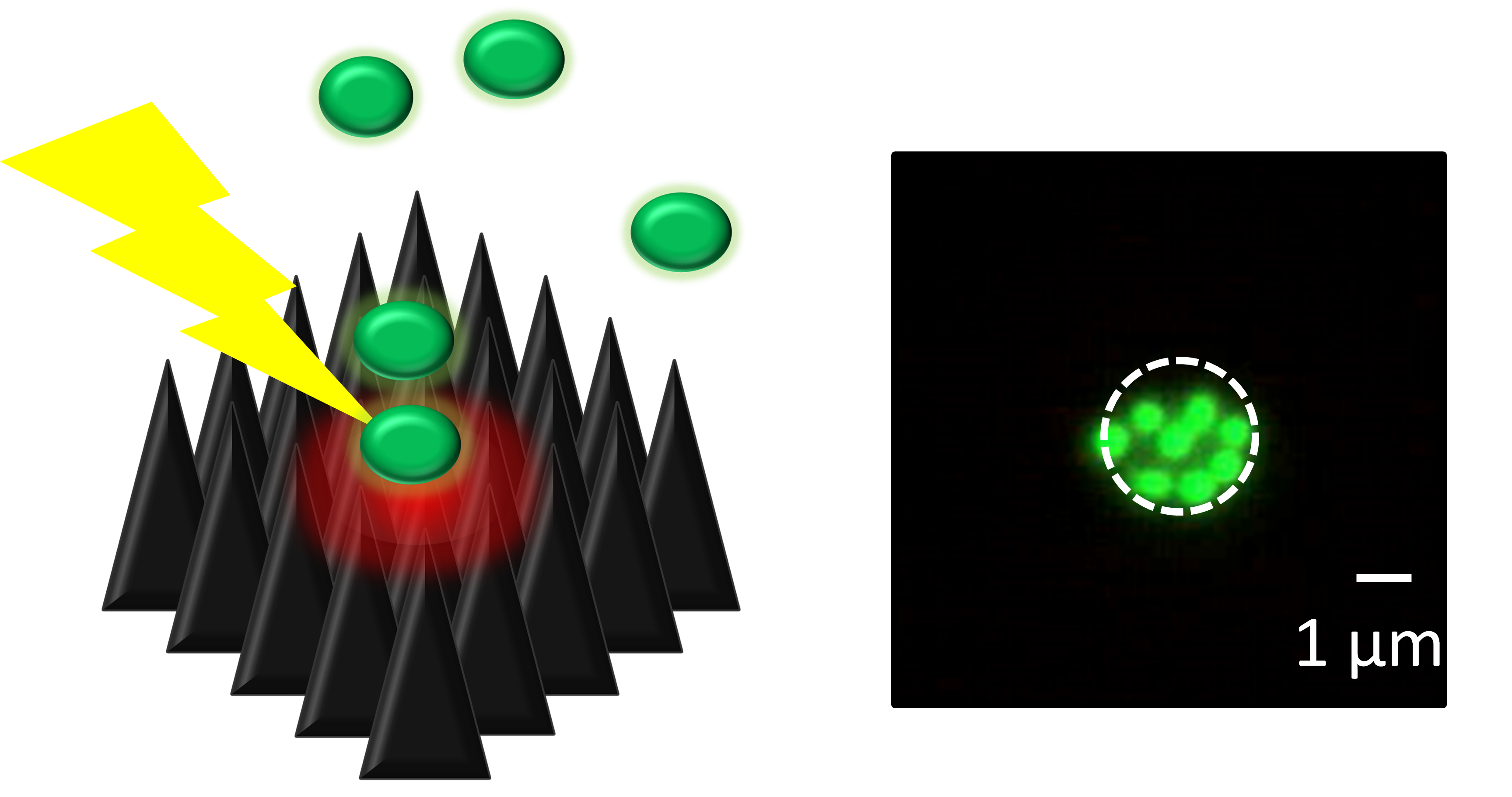
(left panel) Illustration of the concept of NASSCA optical tweezer.
(right panel) Representative micrograph of trapping of nanosphere (latex beads with diameter = 500 nm) by NASSCA optical tweezer.
Yasuyuki Tsuboi
(Planned Research A04, Osaka City University)
In this study, we demonstrated a novel optical tweezer that is potentially superior to plasmonic optical tweezers. It is based on a semiconductor with a nano-structured surface. We call it NASSCA Optical tweezer (Nano―Structured SemiConductor-Asssted optical tweezer). Nowadays, optical tweezers have undergone explosive developments in accordance with a great progress of lasers. In the last decade, a breakthrough brought optical tweezers into the nano-world, overcoming the diffraction limit. This is called plasmonic optical tweezers (POTs). POTs are powerful tools used to manipulate nanomaterials. However, POT has several practical issues that need to be overcome. First, it is rather difficult to fabricate plasmonic nanogap structures regularly and rapidly at low cost. Second, in many cases, POT suffers from thermal effects (Marangoni convection and thermophoresis).
Here, we propose an alternative approach using a nano-structured material that can enhance the optical force and be applied to optical tweezer. This material is metal-free black silicon (MFBS), the plasma etched nano-textured Si. We demonstrate that MFBS-based optical tweezers can efficiently manipulate small particles with characteristic features. The advantages of MFBS-based optical tweezers are: 1) simple fabrication with high uniformity over wafer-sized areas, 2) free from thermal effects detrimental for trapping, 3) switchable trapping between one and two - dimensions, 4) tight trapping because of no detrimental thermal forces, and so on. NASSCA optical tweezer can potentially manipulate various nano^materials.
(1) T. Shoji, T. Tsuboi, 応用物理, 2017, 86, 1, 45-49.
(2) T. Shoji et al. & Y. Tsuboi, Sci. Rep., 2017, 7, 12298.
2017.09.18 Amyloid fibril formation by optical trapping
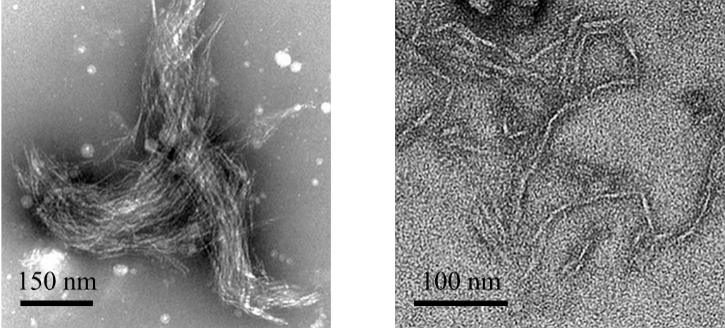
Fig. 1 Amyloid fibrils of cytochrome c formed by optical trapping.
Teruki Sugiyama
(Planned Research A04, Nara Institute and Science and Technology)
Protein amyloids have received much attention due to their correlation with serious diseases such as Alzheimer and Parkinson diseases. In order to develop a new treatment and prevention methods for these diseases related to amyloids, deep understanding of dynamics and mechanism of amyloid formation is indispensable. However, most of the experiments for amyloid formation have been carried out in solution by tuning pH, salt concentration, and temperature and by applying ultrasonication, so that it is quite difficult to predict and control the time and position of amyloid formation, which will prevent us from further investigating its dynamics and mechanism.
In this paper, we demonstrated amyloid fibril formation of oxidized monomeric horse cytochrome (cyt) c, which is a well-known globular heme protein, and its domain-swapped dimer using optical trapping method. Optical trapping of cyt c clusters in solution produced a single assembly consisting of its amyloid fibrils always in the laser focus, thus the amyloid fibril formation was spatially controlled. The resultant assembly was confirmed to show the fluorescence enhancement of ThT and to include the fibril structures by TEM measurement (Fig. 1), indicating that amyloid fibrils are surely produced by optical trapping. In future, we will extend this methods to various amyloid proteins to confirm the wide applicability of this method, and elucidate the dynamics and mechanism of amyloid fibril formation spectroscopically.
(1) K. Yuyama et al., Angew. Chem., Int. Ed., 2017, 56, 6739.
2017.09.07 Photofunctionality of Composition- and Shape-controlled ZAIS Quantum Dots with Less Toxicity
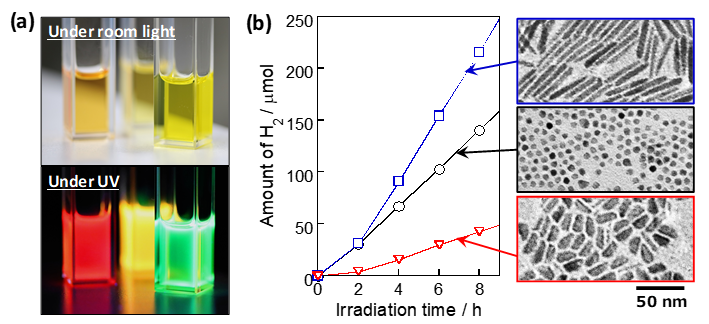
Fig. 1 (a) Photoluminescence of ZAIS QD solutions and (b) photocatalytic H2 evolution of ZAIS QDs with different shapes.
Tsukasa Torimoto
(Planned Research A04: Nagoya University)
Semiconductor quantum dots (QDs) exhibit unique optical properties depending on their size and composition and have been intensively investigated for the applications to display devices, LEDs and bioimagings. In this research project, QDs are also being utilized as target materials for optical manipulation. So far, the QDs composed of groups II-VI semiconductors, such as CdSe and CdTe, have been mostly investigated, but their high toxicity limits the range of their use in practical application. Recently, many efforts have also been made to synthesize I-III-VI2-based QDs composed of only low-toxic elements, being an alternative to the conventional binary QDs with high toxicity. Recently, we have successfully developed highly photoluminescent QDs composed of ZnS-AgInS2 solid solution (ZAIS), in which spherical ZAIS particles of several nanometers in diameter exhibited high PL QY of ca. 80% and the PL color could be widely controlled by changing their particle size and composition (1).
In this study, we report the novel synthesis of anisotropic-shaped ZAIS QDs with precisely controlled composition and their photocatalytic activity for H2 evolution (2). As shown in Fig. 1, ZAIS QDs with well-controlled anisotropic shapes, rod and rice shapes, were prepared by changing the reaction conditions. With irradiation to the ZAIS QDs dispersed in an aqueous solution, the amount of H2 evolved linearly increased. The photocatalytic activity was significantly dependent on the particle shape and chemical composition of ZAIS QDs, in which the optimal activity was observed with rod-shaped ZAIS QDs of 2.6 eV in Eg. Currently, we try to assemble these ZAIS QDs with optical manipulation technologies and investigate the dependence of their photochemical properties on the shape of individual QDs and the array regularity.
In contrast to conventional binary QDs, multinary QDs have unique features, such as the less toxicity and the controllability of electronic energy structure with the particle size and chemical composition. These properties of multinary QDs allow us to design and fabricate novel optoelectronic devices. The research for these QDs become increasingly important from both the fundamental and applied aspects.
(1) T. Torimoto et al., J. Phys. Chem. C, 2015, 119, 24740.
(2) T. Torimoto et al., ACS Appl. Mater. Interface 2016, 8, 27151.